The function of a preamplifier is to allow the user to select a signal from
one or other of a variety of sources--such as a radio, a tape recorder, a
gramophone pick-up or a CD player--to offer an input impedance and signal level
handling capacity which is appropriate to the chosen source, to adjust the
amplitude/frequency characteristics of the input signal, if necessary, to give
the required tonal quality, to amplify the signal, if required, to the 0.5-5V
rms level needed to drive an audio power amplifier, and, finally, to provide
this output signal at an adequately low impedance level.
In earlier, thermionic tube operated audio systems the minimal functions of
a preamplifier were often performed by a simple selector switch and volume
control at the front end of the power amplifier, or perhaps by an additional
tube, with facilities to switch between one or two alternative frequency response
shaping networks. These would be used to provide selective attenuation or gain
adjustments, either by passive RC networks or by negative feedback arrangements.
It was unusual at this period to employ a separate preamplifier unit, so where
more extensive signal manipulation circuitry was provided- as in the case of
the Williamson, or the Brimar preamplifier shown in FIG. 16 - it was usually
incorporated within the main amplifier housing, an arrangement which is now
described as an integrated amplifier. It was not until the advent of the transistor,
with its small size, its low power requirements, its absence of AC operated
heater circuitry and its low heat generation, that the advantages of segregating
the low signal level input circuitry and the high signal level power stages
and power supply wiring could be seen as a normal feature in high quality systems.
It is also required that the signal manipulation and amplification carried
out by the preamplifier shall be done without significantly impairing the distortion
or the signal/noise ratio of the input signal, though the weight attached to
the term significantly will probably be a matter for debate between the designer
and his critics-- who may be insulated by their armchairs from the need, in
practice, to make accommodations between desirable, but not always mutually
compatible, requirements.
A friend of mine, for whom the purchase, use, and subsequent resale of Hi-Fi
exotica appeared to be a major hobby interest, told me that in his experience
there were greater differences in the sound of different preamplifiers than
there were between comparably well-designed power amplifiers. If he was correct
in this belief, it is probable that the tonal differences he had noted had
their origins in the various parts of the circuit in which the gain/frequency
response of the system had been deliberately modified, for example to compensate
for the skewed amplitude/frequency response used in the recording of an LP
or EP gramophone disc.
Gramophone Record Replay Equalization Characteristics
Various combinations of recording pre-emphasis and replay de-emphasis have
been proposed for use in gramophone record reproduction. Of these, the most
relevant is the method recommended in the RIAA:BS1928/1965 specification, in
that this has been adopted, on a world-wide basis, for the manufacture of 33
and 45rpm vinyl discs.
The proposed pre-emphasis characteristics were based on a recognition of the
practical problems in disc manufacture and reproduction using velocity sensitive
(e.g. electromagnetic) cutting and replay heads. These realities also influence
the extent to which electrical headroom is needed- a matter which I will explore
later on. In its original form, the RIAA specification required a replay characteristic
of the form shown in FIG. 1, in which the shape of the replay response was
defined by three time constants, 3180~ts, 318~ts and 75~ts. There has, however,
been a recent amendment to this specification, in acknowledgement of the inevitable
VLF noise present on LP disc replay, to include an additional LF roll-off defined
by a 7950~ts time constant, as shown in the dashed line curve in FIG. 1.
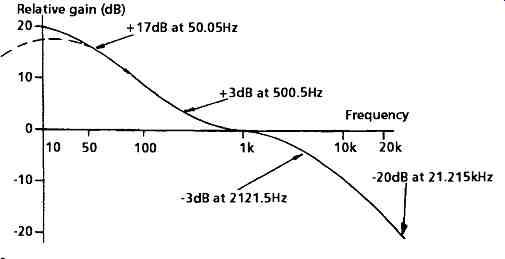
FIG. 1 RIAA response curve
The need for the rising replay gain characteristic below 1kHz is because of
the typical maximum allowable LP/EP groove separation of 0.01 cm, which limits
the magnitude of the permissible recording cutter excursion (to avoid adjacent
groove breakthrough) with a consequent linear reduction in replay stylus velocity
(and output voltage from the pick-up) with decreasing frequency. The concept
of HF recording pre-emphasis, followed by replay de-emphasis, was initially
adopted for 78rpm records, so that the reduced level of treble, on replay,
would lessen the irritating audible hiss due to the emery powder loading of
the shellac discs. This arrangement has been retained even in the case of the
much quieter vinyl surfaces. The most commonly used of the several 78rpm equalization
specifications was similar to the RIAA but with time constants of 3180μs
and 450μs to 1kHz, and then with a fall off in gain defined by a 50μs time
constant to 21 kHz.
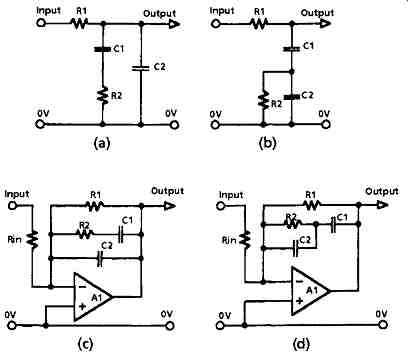
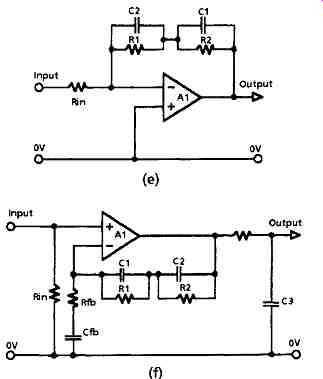
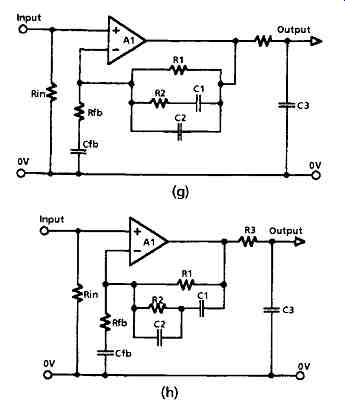
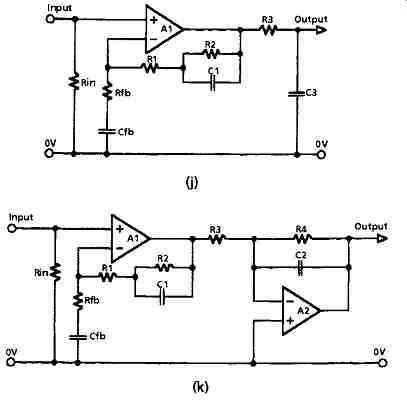
FIG. 2 RIAA replay circuits
Replay Equalization Circuitry
There are a number of circuit arrangements which will generate the required
RIAA replay response curve for use with velocity sensitive gramophone pick-up
transducers, and I have shown the more commonly used circuits in FIG. 2. Of
these, the simplest, and therefore, in the opinion of the purists, the most
desirable options are the two passive networks shown in FIGs 2a and 2b. However,
in order for these to function correctly they must introduce an attenuation
of 20dB at 1kHz, and this will require some additional amplification to restore
the original signal level. Also, for the frequency response of the networks
to conform accurately to the RIAA curve, it is necessary for them to be driven
from a very low impedance source and for the output load to offer an impedance
which is high in relation to R1. In practice, these requirements imply the
need for further amplification or buffer stages preceding or following the
passive network, so the presumed advantages of simplicity and absence of (possible
distortion introducing) active components are lost.
The layouts of FIGs 2c, 2d and 2e can be arranged so that they can be fed
directly from the pick-up cartridge, with Rin chosen to provide the manufacturers
specified loading for the cartridge. This layout also offers a low output impedance
to any following stages which avoids the need for additional pre- and post-amplification.
All three of these circuits use shunt feedback, which has the relative disadvantage
that the input thermal noise is determined by the source impedance in series
with Rin , which will be worse, at low to mid-frequencies with an inductive
transducer, than that given by a equivalent series feedback layout. A simple
calculation would suggest that the source noise level for a series feedback
circuit, such as that of FIG. 2g, when used with a pick-up cartridge having
a DC winding resistance of 2200 ohms (connected in parallel with a 47,000 ohms
load resistor) will be 0.83gV. (This figure is the room temperature value seen
at the input of a feedback circuit of the kind shown in FIG. 2g, with a 20Hz-20kHz
measurement bandwidth.) The calculated value for a shunt feedback system, of
the kind shown in FIG. 2e, with an input (pick- up load) resistor of 47,000
ohms, will have a noise voltage of 4.24uV under the same measurement conditions.
However, these figures are modified by the fact that the measurement bandwidth
for an RIAA equalized gain stage is not 20kHz, but less than a tenth of that
figure, which changes the noise output figures to 0.24gV and 1.22gV respectively.
Also, pick-up cartridges will have a significant value of inductance - the
quoted value for a Shure M75 cartridge being 450mH -- and this will increase
the source noise voltage at the upper end of the audio pass-band. The effect
of this is to alter the relative character of the noise given by these two
systems, so that the noise from a series-type circuit is a high pitched hiss,
while that from a shunt-type circuit is more of a low pitched rustle.
There are also a few other advantages offered by shunt feedback RIAA circuits
such as those shown in FIGs 2c, 2d and 2e. Of these, the most important, in
practice, is that they are largely immune to very short duration input overload
effects, which can be caused by voltage spikes in the cartridge output arising
from dust and scratches on the surface of the record. These short duration
overloads can greatly exaggerate the clicks and plops which are such a nuisance
on vinyl disc replay. Also, they have, in theory, a lower level of distortion
(see Taylor, E.F., Wireless World, April 1973, p. 194) -- although this is
a matter only of academic interest in the presence of the 0.5-1% THD generated
at 1kHz by a typical pick-up cartridge ( Hi-Fi News and Record Review, October
1982, pp. 59-68). Shunt feedback layouts can also give a precise match to the
RIAA specification, which a series feedback circuit, as it stands, will not.
This is because any series feedback layout having a feedback limb which decreases
in impedance with frequency will tend to unity gain at high frequencies, whereas
the RIAA specification stipulates a gain curve which tends to zero. Unfortunately,
in many preamplifiers of commercial origin, which use series feedback RIAA
stages in order to obtain the lowest practical (input short-circuited) noise
levels, this error is ignored, even though its effect can easily be seen on
an oscilloscope ( Wireless World, October 1982, pp. 32-36) and the tonal difference
which it introduces can equally easily be heard by the listener.
This error has been corrected, in the case of the circuit layouts shown in
FIGs 2f, 2g and 2h, by adding a final RC lag network, R3/C3, in which the required
component values will depend on the gain of A 1 at 20kHz. The gain reduction
in the 1kHz-21kHz part of the pass-band required by the RIAA spec. can be made
more simply by dividing the circuitry used to provide this response into two
parts- as in FIG. 2j - where R1, R2 and C1 generate the frequency response
curve from 30Hz to 1kHz and R3 and C3 provide the 75us time constant needed
for the 1kHz-21kHz part of the spectrum. A possible drawback with this circuit
(which will also apply to any final frequency response correction lag network),
depending on the nature of the following circuitry, is that the time constant
of R3/C3 will be affected by the circuit load impedance, which is, for the
purposes of this calculation, in parallel with R3. This difficulty is removed
by the use of an active output integrator, shown as A2 in FIG. 2k, where R4/C2
provide a 75us time constant. The only likely problem with this arrangement
is that the circuit introduces a phase reversal, which may not be desired.
The required component values for these networks were originally calculated
by Livy and quoted in an article describing a simple RIAA equalizing stage
(Livy, W.H., Wireless World, January 1957, p. 29) shown in FIG. 3a. The calculations
were recast by Baxandall in a slightly more accessible form, and quoted in
a reference work (Baxandall, P.J., Radio, TV and Audio Reference Book, S.W.
Amos, ed., Newnes- Butterworth, Section 14), in the course of an excellent
review of RIAA equalization options.
Using the formulae quoted by Baxandall, the required values for R1, R2, C1
and C2 in FIGs 2a, 2c and 2g can be determined by:
R1/R2 =6.818 C1 R1 = 2187us C2 R2 = 109us
For FIGs 2b, 2d and 2h the component
values can be obtained from:
R1/R2 = 12.38 C1 R1 = 2937us C2 R2 = 81.1us
Similarly, in FIGs 2e and 2f, the values are given by:
R1/R2 = 12.3 C1 R1 = 2950us C2 R2 = 78us
Practical Circuit Layouts
In tube amplifiers the most common RIAA equalization circuitry was of the
kind shown in FIG. 3a, due to Livy, in which the frequency dependent feedback
network is connected between anode and grid of a high gain amplifying tube-
typically a pentode of the EF86 type. Translating this type of circuitry into
a transistor form using a single bipolar junction transistor (see Tobey, R.,
and Dinsdale, J., Wireless World, December 1961, pp. 621-625, and also Carter,
E., and Tharma, P., Wireless World, August 1963, pp. 376-379), encountered
the two problems that the input impedances were much lower than was the case
with a tube amplifier and that the available gain was inadequate to achieve
the required frequency response from the stage, especially at the low frequency
end of the RIAA curve where a gain of +20dB with respect to that at l kHz was
needed. The normal approach to this problem was to increase the value of R1,
which increased the LF gain at the expense of accuracy in conforming to the
lower end of the RIAA curve.
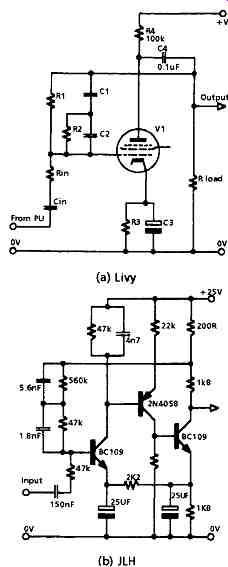
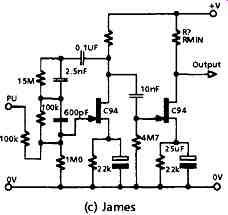
FIG. 3
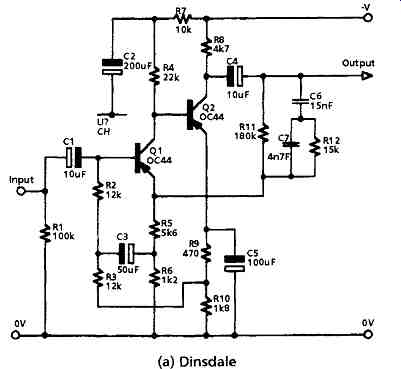
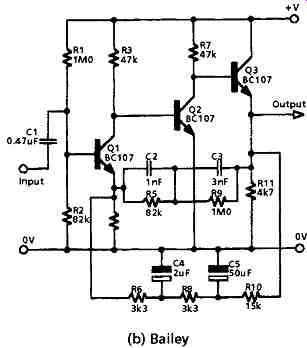
FIG. 4 Further RIAA stages
In a rather later circuit of my own ( Wireless World, July 1969, pp. 306-310),
shown in FIG. 3b, I elaborated the single transistor circuit into a triplet,
which greatly increased the available gain and also allowed a third order (18dB/octave),
30Hz high pass rumble filter to be implemented using the negative feedback
path between the two BC 109 emitters.
The availability of junction FETs, which approached much more closely in their
operating characteristics to the thermionic tube- with the exception of a much
lower gain - encouraged their use in circuits based on their tube precursors,
and an FET based design due to James, using some early devices of this type
(James, D.B.G., Wireless World, April 1968, p. 72), is shown in FIG. 3c.
The circuit due to Dinsdale (Dinsdale, J., Wireless World, January 1965, pp.
2-9), shown in FIG. 4a, was one of the earliest of the transistor based RIAA
equalization circuits to use series feedback around a gain block having a useful
amount of stage gain, and became the standard design adopted by a large number
of Hi-Fi manufacturers, though with silicon planar transistors rather than
the germanium diffused junction types used by Dinsdale. A problem with this
design was that the equalizing network applied a capacitative load on the output
of Q2, which reduced the gain of Q2 at the upper end of the audio spectrum,
although, in practice, this might go some way to remedying the inadequate HF
attenuation which is characteristic of the series feedback RIAA system. This
problem of falling HF gain was remedied by Bailey (Bailey, A.R., Wireless World,
December 1966, pp. 598-602) by the use of a transistor based version of the
tube Ring of Three shown in FIG. 4b. This had a low impedance, emitter-follower
output stage, and also incorporated a second-order high pass rumble filter,
via R6-R10, to attenuate signals below 30Hz.
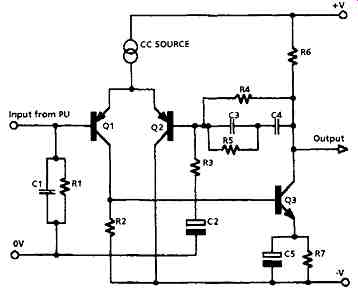
FIG. 5 Direct coupled RIAA stage
With the growing use of symmetrical +/- power supply lines, the discrete component
version of the RIAA stage shown in FIG. 5 has become popular, either in the
form shown, or in its complementary version, and usually with some elaboration
of the general structure. For a simple design with normal bipolar junction
transistor inputs, PNP input devices will normally be chosen because of their
better noise performance, although this design decision will be influenced
by the availability of application specific components such as the NPN super
match pairs described below. This circuit arrangement illustrates one aspect
of modem design practice, which favors DC coupling to minimize the use of capacitors
in the signal path (except when their use is forced, as, for example, in the
RIAA frequency correction network). If Q1 and Q2 were a matched pair it would
be possible also to dispense with the DC blocking capacitor (C2) in the feedback
line. The capacitor, C1, across the pick-up load resistor will have a value
recommended by the cartridge manufacturers to obtain the flattest possible
frequency response.
Most contemporary RIAA equalizing circuits are based on versions of FIGs 2g
or 2h, though there is an increasing trend to the use of versions of FIGs 2c
and 2d in which the output from the pick-up cartridge is amplified by a low
noise, fiat frequency response gain stage, and then presented to the RIAA stage
at a signal and impedance level at which the noise level differences between
shunt and series connected NFB networks are insignificant. The differences,
at the high quality end of the market, between one manufacturer's products
and another, now relate to whether integrated circuit or discrete component
gain blocks are used as the amplifying stages, and, if the latter, the type
of component layout chosen.
Very Low Noise Input Systems
Towards the end of the vinyl era -- to the great regret of many gramophone
record users, the manufacture of the 12 inch LP disc was discontinued in the
late 1980s by the major record manufacturers in favor of the more robust and
less expensive compact disc--the phono pick-up cartridge design which
had come to be preferred by the connoisseurs was one using a moving coil type
of construction, rather than the moving magnet or variable reluctance style
of mechanism which had dominated the high quality end of the audio market for
the previous forty years. The lower mass of the moving coil transducer, and
its more effective linkage to the stylus assembly allowed an improvement in
the dynamics and stereo channel separation of the system, and the much lower
inductance of the pick-up coils greatly improved its high frequency response.
However, the typical mean signal level output voltage from such a cartridge
might well be only some 50-500uV rather than the 3-5mV output voltage which
would be given by a moving magnet transducer, and this required a different
approach to RIAA equalized input stage design.
Moving coil pick-up cartridges had been manufactured for many years before
there were suitable components for the construction of electronic head amplifiers,
and the necessary amplification was provided by an input step-up transformer
of the type which had been employed for the same purpose with low output-voltage
microphones.
However, the use of an electronic gain block offered advantages in terms of
wider bandwidth and greater freedom from unwanted hum pick-up.
The main approach employed by the audio circuit designers was to place this
gain block between the pick-up cartridge output and the input to the RIAA stage.
This way was chosen because it was easier at that time to design a flat frequency
response head amplifier than to make a switched gain RIAA stage with a noise
level which was fifty times lower than the current norm. For such a gain block
to have such a low input noise level it would need a lower input circuit impedance
than would be found in a normal small-signal transistor amplifier. There are
various ways by which this could be achieved. Of these, the most obvious was
to connect a number of input devices in parallel, as shown in the circuit of
FIG. 6, due to Naim. Here five transistors have been arranged in this way,
which would, other things being equal, reduce the input base spreading resistance
(that distributed resistance between the base connection on the chip and the
active base region of the transistor) to one fifth, and reduce the input noise
voltage to 1/~/5 or a little less than a half. Some of this input noise improvement
is lost, unfortunately, because of the need to include additional emitter resistors
to ensure current sharing between the unmatched input devices.
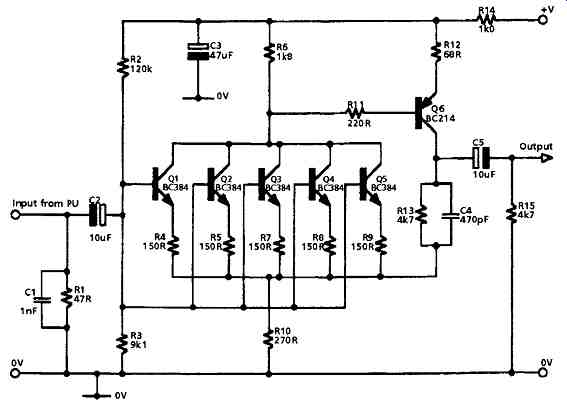
FIG. 6
Naim NAC 20 head amp
Another approach, rather more suited to the DIY constructor than the large-scale
manufacturer, is to use a small-power transistor as the input device, because
these will have a larger chip size and, in consequence, a lower base spreading
resistance.
However, the use of such input transistors might require a degree of selection
to find those having the best noise level. An example of this type of circuit
is shown in FIG. 7, for a 30/60 ~ head amplifier, particularly suited to very
low impedance, low output voltage cartridges such as the Ortofon MC20. In this,
Q1, a BD435 small- power transistor, is operated as a grounded base amplifier
with a load resistor (R3) which is bootstrapped to increase the stage gain,
while NFB is applied via R7, R4 and R5. The output impedance at Q1 collector
is reduced to a low level, as seen at the output, by Q2 and Q3, which are connected
as a high gain, compound emitter-follower. The circuit is designed to be powered
from a pair of 1.5V AA cells, from which the current drain is 1.5mA/channel.
The -~ 1 dB bandwidth is 10Hz-40kHz, and the THD is less than 0.02% at 1V output
at 1kHz.
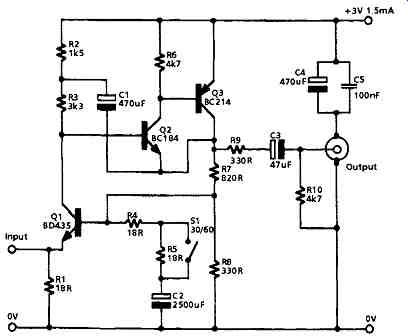
FIG. 7 Grounded base head amp
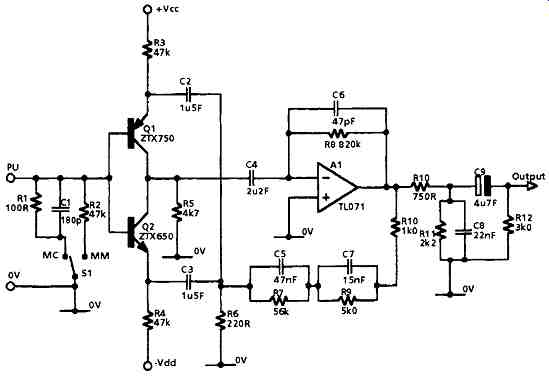
FIG. 8 Quad M~MM input circuit
The other way in which the input resistance of the amplifier may be reduced
is by operating the input devices in push-pull, which will double the gain
and halve the effective noise resistance. An elegant way of doing this is that
used by Quad, and shown in FIG. 8. This takes advantage of the fact that a
silicon bipolar junction transistor can operate as an amplifier, though admittedly
only at relatively low input signal levels, when its collector voltage is no
greater than that on its base. Operation at such a low collector voltage will
help to reduce the shot noise component due to collector-base leakage currents
in the input devices. Quad used this layout in all their pre-1994 RIAA input
stages, with component values being chosen to suit their use either as moving
magnet or as moving coil input arrangements. Although the main op amp gain
block, A1, is operated in the shunt feedback mode, the circuit as a whole
uses series FB because of the interposition of Q1 and Q2. R10, R11 and C8
provide the necessary additional HF attenuation to correct the inevitable series
FB gain error.
Low Noise Devices
Bipolar junction transistors
The concept of using multiple, parallel-connected, input transistors as very
low noise input devices offered an opportunity to the IC manufacturers to use
their existing fabrication techniques to make low noise matched pair transistors
where each transistor was, in reality, a large number of parallel-connected
devices - distributed across the face of the chip to average out their characteristics.
The National Semiconductors LM194/394 devices were early examples of this type
of construction, and offered input noise resistance figures of the order of
40 ohms, and bulk (emitter circuit) resistances of about 0.4 ohms. Recently,
Precision Monolithics have offered their MAT-01 and MAT-02 super-match pairs,
and their SSM-2220 and SSM-2210 equivalents, which are respectively NPN and
PNP matched dual transistors, which have input noise resistance values less
than 30 ohms. An ultra-low noise gramophone pick-up input head amplifier stage,
based on the SSM-22 l0 and SSM-2220 super-match pairs, is shown in FIG. 9.
This circuit makes quite an interesting design study, with the Q2 pair of
transistors acting as a cascode load for Q1 to increase the input gain, with
the Q3 pair acting as a current mirror to sum the outputs of the two halves.
Because the bases of Q2 are held at approximately 3V, the collector voltages
for Q1 are clamped at about +2.4V, which offers adequate input headroom while
still being low enough to minimize collector- base leakage currents. Constant
current sources form tail loads for Q1 and collector loads for Q4. In addition
to increasing the stage gain, this assists in rejecting signal breakthrough
.from the power supply rails. Switch S1 allows a choice of input resistances
and a choice of stage gain levels, 15 or 150", to suit MM and MC pick-up
inputs.
Junction FETs
Very low noise JFETs have been available for at least ten years from specialist
manufacturers such as Hitachi, Siliconix and Intersil, and, more recently,
have also been readily obtainable in dual matched pairs, specifically intended
for use in amplifier input stages. The availability of FETs in matched pair
forms avoids two of the problems inherent in these devices; that the drain
current vs. gate voltage relationship is influenced by so many factors, such
as doping level, channel geometry and the distribution of doping impurities,
that their drain current cut-off voltage, [Vgs(off) ] and their drain current
at zero gate voltage (IDss) are very variable from one device to another, so
that a considerable degree of selection would be necessary to obtain a balanced
pair.
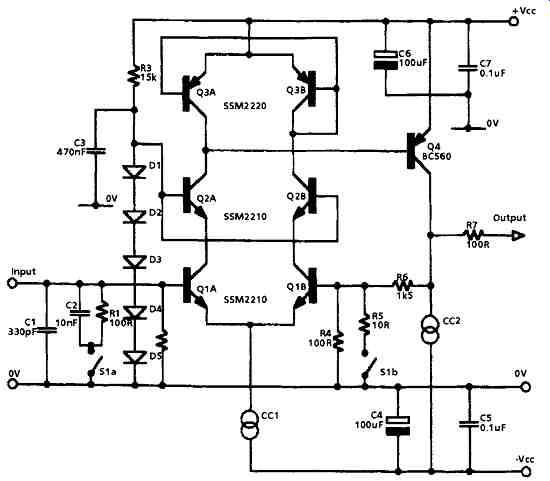
FIG. 9 Super-match pair head amp
In terms of noise levels, JFETs traditionally suffered from a higher level
of flicker (I/f) noise than junction transistors. Also their channel resistance
is much higher- because of their method of construction- than those of BJTs.
Improvements in 1/f noise have been made by greater care in their physical
construction and the channel resistance has been lowered by connecting a large
number of channels in parallel, in a similar way to that used in making a junction
transistor super-match pair.
A very high performance RIAA corrected input stage, due to Spectral Inc.,
is shown in FIG. 10. This also has a cascode connected input stage but with
the bias offset voltages of Q2a and Q2b used to provide the 2-3V drain voltage
required by Q1a and Q1b. Q3a/Q3b provide a second gain stage, with Q4a and
Q4b forming a current mirror collector load for Q3. A symmetrical emitter-follower
pair is used to lower the preamplifier output impedance, to allow it to drive
a low resistance RIAA equalizing network in the feedback path to Q1b. As is
current Hi-Fi practice in the USA, aimed at lowering LF phase errors, there
are no DC blocking capacitors in the signal path, so RV1 is used to trim out
any small residual DC output offset.
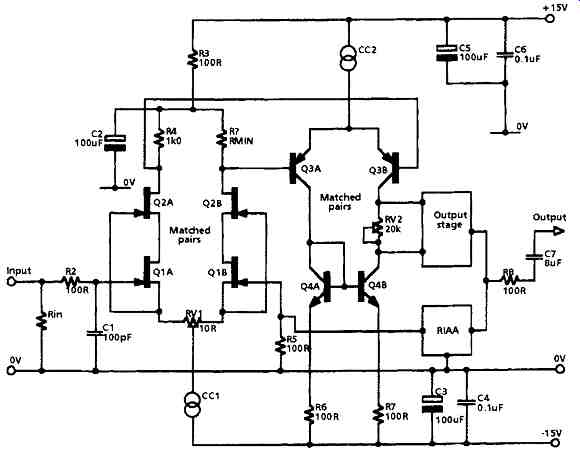
FIG. 10 Spectral RIAA input stage
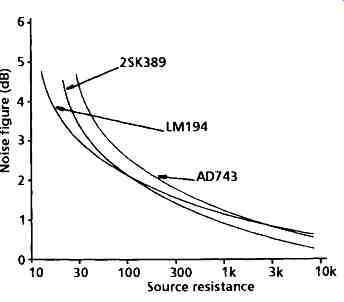
FIG. 11 JFET/BJT noise figures
The input noise resistance of an FET, at drain voltages which are low enough
to avoid significant drain-gate leakage currents, is determined principally
by the resistance of the channel, and this is related to the slope (gfs) of
the drain current/gate voltage curve, in an ideal device, by the equation:
R(n) = 0.67/gfs (ohms)
As an indication of the relative performance of contemporary JFET and bipolar
transistors, the noise characteristics of LM194 and 2SK389 monolithic matched
pairs are shown in FIG. 11. This shows that, for input circuit resistances
of above 100 ohms, the 2SK389 FET has a lower noise figure than the best of
the bipolar devices.
Low noise op amps Inevitably, all improvements in device manufacture, as applied
to discrete components, will find their way into the field of IC manufacture,
and this results, in the present case, in the availability of audio type ICs,
such as the Analog Devices AD743 bifet op amp, which has a 10kHz noise voltage
of 2.8nV/~/Hz and an output distortion figure of 0.0003% at 1kHz and 5V rms.
I have also plotted the noise figure of the AD743 on FIG. 11, on which it appears
little worse than that of the 2SK389. Under the circumstances, there seems
to be little point in using elaborate discrete component circuits such as those
shown in FIGs 9 and 10, as an alternative to an IC, except to provide greater
headroom than that would be available from an IC op amp operating from 15V
supply rails.
Headroom
Very few topics can have generated as much debate in the audio field as the
extent to which the designer should allow for possible input overload in any
given circuit, a factor generally referred to as headroom. Sadly, much of this
debate has been entirely misguided. The reason for this is that all the sources
which provide signals to the preamplifier do have specific output voltage limits.
For example, in the case of a tape recorder, either reel-to-reel or cassette
type, the recording level is generally chosen so that the peak output signal
is not more than 3dB (~/2") greater than the normal span of the recording
range. At +6dB, the output THD would probably have increased, in the case of
a cassette recorder, from about 0.5% to some 3-5%, and at +12dB (4 ")
magnetic saturation of the tape would probably have clipped the peaks of the
signal anyway, preventing any further output voltage increase.
In an FM tuner, constraints on the permissible frequency excursions of the
broadcast signal impose stringent limits on the magnitude of the modulation,
and this is rigidly peak limited before being broadcast- again giving a +6dB
maximum likely signal over-voltage limit. In the case of CD the recording engineer
will want to use the bulk of the available 16-bit (65,536) output levels,
while there is an absolute prohibition on any attempt to go beyond this, so,
once again, there is an output voltage ceiling, which is unlikely to be greater
than +6dB above the intended maximum recorded level.
This leaves only the vinyl gramophone disc as a source of possibly unlimited
peak output voltages, but, here again, at least for recorded signals, there
are output level constraints, as shown in FIG. 12. The recording characteristics
of the vinyl disc were analyzed by Walton (Walton, J., Wireless World, December,
1967, pp. 581-588), to whom I am indebted for the data on which FIG. 12 is
based. Below about l kHz, the electrical output from the pick-up cartridge
is limited by the allowable 0.01 cm spacing between the grooves, and the resulting
0.005cm maximum permitted cutter excursion. Above a turnover frequency of around
1kHz the possible closeness of succeeding groove excursions, which control
the output voltage, is determined by the shape of the rear face of the cutting
head and the linear velocity of the stylus in the groove (which will depend
on the track diameter). Beyond about 3kHz there is a limit imposed by the maximum
groove accelerations which the cutter head can generate or the pick-up stylus
can follow. These are also limited by the groove velocity, and thus depend
on the track diameter.
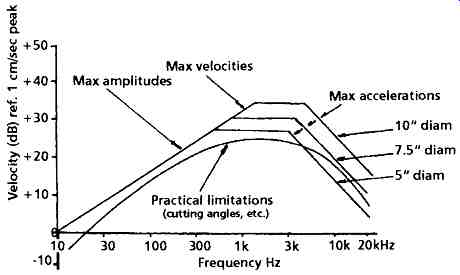
FIG. 12 Maximum recorded levels.
Walton considered that, under optimum conditions, and at a 7.5" groove
diameter, a groove modulation velocity equivalent to +30dB at 2kHz, with respect
to 1 cm/s, was the maximum which would be cut. The Shure Corporation, which
made a specialty of the manufacture of pick-up cartridges with very high record
groove tracking ability, claimed that its best models were capable of following
a groove modulation of +40dB ref. 1 cm/s at 2kHz, decreasing with increasing
frequency. They obviously considered that the musical bells track on their
stereo test record, which was cut at a recording level of +25dB at 10kHz, would
present a formidable challenge to their competitors- a consideration which
lends support to Walton's curve of practical output limits in FIG. 12.
From this analysis, if a recorded velocity of 5cm/s is taken as the normal
maximum mid-band signal, the limitations of the recording process will ensure
that the maximum modulation velocity which the pick-up will encounter on a
very heavily modulated part of the recording will not exceed some 30cm/s. This
practical limitation on the pick-up cartridge output voltage was also shown
by Wolfenden (Wolfenden, B.S., Wireless World, December 1976, p. 54) using
data from Shure, and by Kelly (Kelly, S., Wireless World, December 1969, pp.
548-555) who had confirmed, by experiment, the magnitude of any feasible overload
margin.
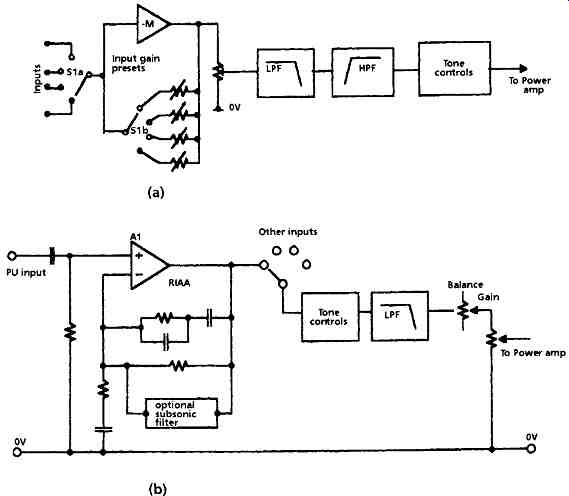
FIG. 13 Basic preamp layouts
However, with the same kind of logic which persuades ordinary, sensible motorists
that a car which is capable of 150mph is a much better proposition- in a country
where the speed limit is 70mph- than one which will only do 90mph, many circuit
designers consider it necessary to provide RIAA stage voltage overload margins
of 20-30", or greater. Unfortunately, by praising such achievements,
reviewers perpetuate the belief that this style of design is both good and
necessary. Clearly, some type of gain switching is necessary if the preamp
is to cope with the voltage output levels from both MM and MC type pick-up
cartridges, but these cartridges will require switch selection of their load
resistance anyway, between, say, 47k and 100R, so the provision of a switchable
choice between low impedance/high gain, and high impedance/low gain options
has become normal practice. In reality, the highest output voltage peaks are
likely to be those associated with dust particles, scratches and other blemishes
on the surface of the disc, and while it is obviously desirable that such sudden
input voltage excursions do not lead to a prolonged paralysis of the amplifier,
clipping which is very brief in duration may be acceptable to the listener.
A typical op. amp gain stage, fed from a +15V supply, is capable of a 9.5V
rms undistorted output voltage swing. It should therefore be capable of handling,
without clipping, the signal from any disc replay amplifier whose normal maximum
output level is less than 1.5V rms. For the greatest freedom from signal overload,
the gain control should be placed as far forward in the signal chain as possible,
as shown in schematic form in FIG. 13a. Unfortunately, with this layout the
total noise contributions from the following stages will be present all the
time, regardless of gain control settings. The alternative option, shown in
FIG. 13b, is to place the RIAA equalization stage between the pick-up input
and the input selector switching. Any tone controls or filters, if used, can
then be based on unity gain blocks, which if they use the same type of gain
stage (such as a low noise op amp) as that used in the RIAA module itself,
will not overload if the RIAA module does not. With this type of layout, the
preamplifier noise contribution will decrease to zero as the signal level is
reduced.
Tone Controls and Filters
In the earlier days of audio, it was taken for granted that there would be
shortcomings both in the tonal quality and in the signal to noise ratio of
all of the input signals. There would also be deficiencies in the frequency
response characteristics of both the input transducers and the loudspeakers,
and it was expected that any high quality preamplifier unit would offer comprehensive
facilities to allow correction of the electrical output signal. However, there
has been a continuous improvement in the quality of the input signal, whether
obtained from compact disc, LP record, FM tuner or cassette tape. Similarly,
there has been a continuing improvement in LS performance, so that, for example,
the bass output is only limited by the physical dimensions of the LS cabinet,
or of the listening room, and additional bass boost would be pointless. Faced
with this situation, many amplifier manufacturers no longer offer anything
other than fiat frequency response amplifier units.
While the flat frequency response approach is probably adequate for most purposes,
I feel that there are still a few remaining situations where, for example,
high pass rumble filters are useful- such as when a new CD has been mastered
from an archive source, and contains significant amounts of LF noise from mechanical
sources, or where a blameless recording suffers from low frequency traffic
noise intrusions into the notionally silent recording studio - or where the
tonal balance chosen by the recording engineer differs from that preferred
by the listener. In this case some form of frequency response tilt control
would probably be useful. I have therefore shown two design examples of these.
The first of these, due to Quad, is shown in FIG. 14, and the second, due to
Bingham (Bingham, J., Hi-Fi News and Record Review, December 1982, pp. 64-65)
is shown in FIG. 15. I have also shown the layouts for four steep cut filter
circuits in FIG. 16.
The filter circuits shown in FIGs 16a and 16b use my own bootstrap design
( Electronic Engineering, July 1976, pp. 55-58) and give a -18dB/octave frequency
response. That of FIG. 16a is a 50Hz high pass layout, and that of 16b is a
10kHz low pass circuit. The filter circuits shown in FIGs 16c and 10.16d have
the same cut-off frequencies, but use the Sallen and Key layout (Sallen, R.P.,
and Key, E.L., IRE Trans. Circuit Theory, March 1955, pp. 40--42) which gives
a-12dB/octave response. For both filters the turnover frequency is given by
the formula:
FIG. 14 The 'Quad' slope control
The turnover frequencies can be changed, up or down, by proportional alterations
to the resistor or capacitor values. For example, if the values of C1, C2 and
C3, in FIG. 16a, are increased by the factor 5/3, the turnover frequency of
this filter will be reduced to 30Hz. Similarly, if the values of the resistors,
R1, R2 and R3, are halved, the turnover frequency of FIG. 16b will be increased
to 20kHz, and so on.
Since the bulk of modem signal sources are not much in need of remedial treatment,
I have not included technical descriptions of the wide variety of tone control
arrangements which have been evolved over the years. For those seeking further
information on these, I would recommend my book Audio Electronics, (Butterworth-
Heinemann, 1965, Section 4, pp. 169-189), or an earlier article of mine in
Electronics World + Wireless World (July 1990, pp. 636--639).
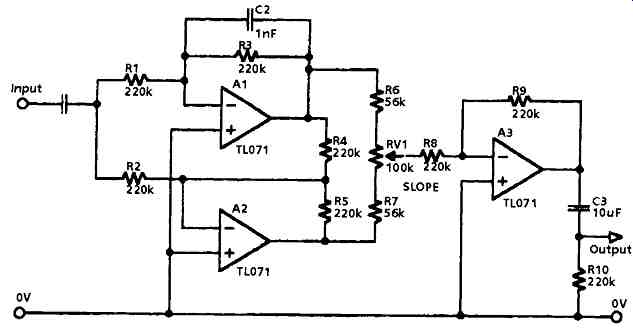
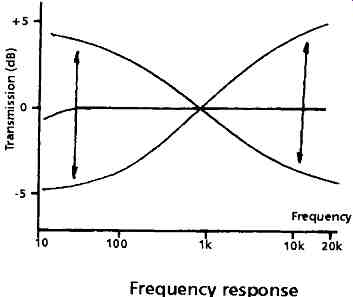
FIG. 15 Bingham's tilt control
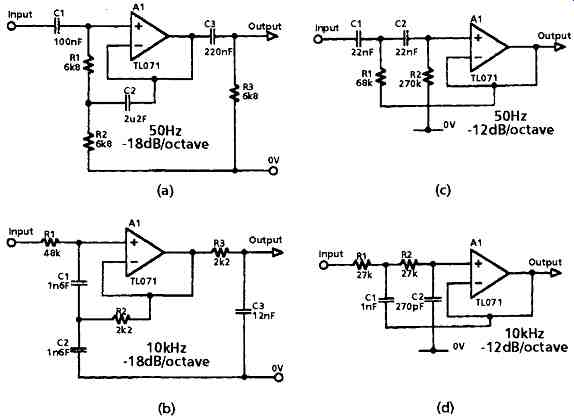
FIG. 16 Steep cut filter circuits
Signal Channel Switching and Remote Controls
The choice between input channels can be made by purely mechanical means,
such as a simple rotary switch or a suitable push-button sliding contact arrangement.
The only normal difficulty with this system is that the knob or the push-buttons
which control the channel selection will be on the front of the preamp box,
while the signal inputs between which the electrical choice is to be made all
go to sockets on the back face of the box, so that the internal connecting
wires which join the one to the other must pass over other parts of the signal
circuitry, and may need to be well screened. If the switching element is a
relay (preferably sealed, and inert gas filled), or a suitable semiconductor
switch, of the kind I have shown in FIG. 17, the input switches can be located
close to the input sockets. It will also allow channel selection to be made
by an external voltage, and this will allow the convenience of remote control,
perhaps by the use of an infra-red transmitter/receiver assembly of the type
widely used for similar purposes with TV sets.
Most of the plain on/off types of signal switching, based on discrete components
or CMOS analogue switches, of the type I have shown in FIG. 17 offer acceptably
low distortion characteristics up to, say, a 1V rms signal level. In the case
of the series connected FET switch, shown in FIG. 17c, the value of Rin should
be high in relation to the 'on' resistance of the FET to swamp any signal induced
changes in the channel resistance. In the case of the CMOS analogue switches
shown in schematic form in FIG. 17d, these devices normally are designed to
operate with logic level input control voltages, and do not significantly distort
input signals so long as their peak-to-peak values lie comfortably between
the limits set by the DC supply rails. The extent of the distortion introduced
by these devices depends on the output load (which should, ideally, be very
high) and on the quality (and cost) of the component itself.
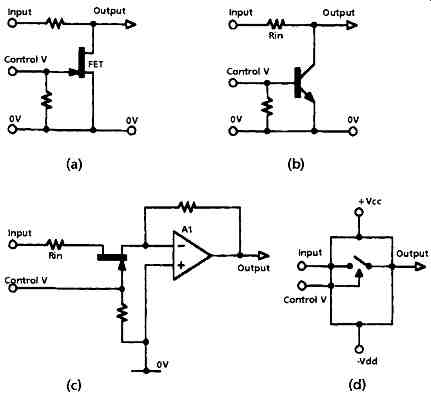
FIG. 17 Voltage controlled switches
Voltage Controlled Gain Systems
While there are a number of low distortion switching circuits available, the
techniques proposed for a continuous, voltage controlled, alteration of the
amplifier gain are usually much less satisfactory. For simple, low cost signal-level
control the FET arrangement of FIG. 18a, in which the FET and the input resistance,
R1, form a simple resistive attenuator, is fairly widely used. As it stands,
this would have a THD figure, at 1V rms and 1kHz output, of some 10-15%. In
the Siliconix file note, AN73-1, it is proposed that negative feedback should
be derived from the drain circuit, by way of R2 and R3 (Siliconix suggest that
the THD is lowest when R2 = R3 10(R1//Rds//RL) ). This will reduce the THD
to about 1-1.5% and although this figure decreases as the signal level is reduced,
it is still very much higher than would be acceptable in any Hi-Fi context.
A commercially available alternative to the discrete component (e.g. FET)
voltage controlled attenuator is marketed as the Motorola MC3340P IC, a device
which was widely used in TV remote control systems. This uses two long-tailed
pair circuits in which the gain is controlled by the tail current. By connecting
the non-inverting and inverting outputs in antiphase the second harmonic distortion
is partially cancelled.
The claimed performance offers a 90dB possible attenuation ratio, a bandwidth
of 1MHz, and a THD of 0.2%, worsening to 3% as the attenuation is increased.
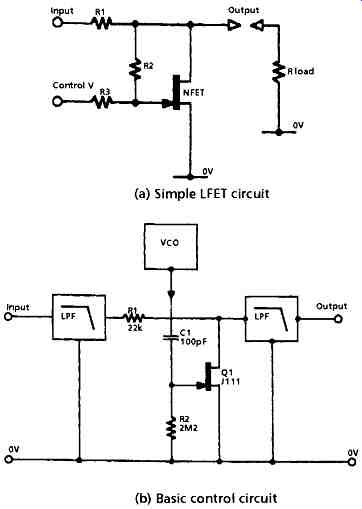
FIG. 18 Attenuator circuits (a) Simple LFET circuit (b) Basic control circuit.
I described the various other available techniques in an earlier article (
Electronics World + Wireless World, April 1995, pp. 320-321), and suggested
the use of a very low distortion discrete component design (intended for use
in a dynamic range control circuit ( Electronics World and Wireless World,
November 1995, pp. 938-940). This gain control circuit was based on a switch-mode
attenuator, shown in schematic form in FIG. 18b. In this circuit a high frequency
(180kHz) rectangular voltage waveform is used to control the proportion of
the time in which the channel is open. This, in turn, is controlled by the
'on to off' ratio of the switching waveform, which can be changed, within the
range 5% : 95% to 95% : 5% by a DC input voltage to the waveform generator
circuit. This gives an attenuation ratio of about 30dB, and a THD- at 1V rms
and 10Hz-10kHz- of less than 0.005%, mainly due to the steep-cut low pass filters
used to remove signal components within 20kHz of the switching frequency.
In their application note AN-105, PMI propose an improved version of the system
used by Motorola, of which I have shown the circuit in FIG. 19. The THD introduced
by this circuit depends on the cancellation of the distortion, mainly second
harmonic, introduced by the controlled transistors, Q l-Q4. For this to be
adequate, there must be an exceedingly close match between all four transistors,
and PMI show this as an application for their MAT-04 monolithic transistor
array. PMI claim a THD value of <0.03% at 3V rms output, for this circuit.
Inevitably, such a useful discrete component layout will be produced in due
course as an IC module, and this has been done by Analog Devices, Inc., in
their SSM-2108T and SSM-2118T ICs, which have a claimed THD in the range 0.013-0.006%
at 3V rms depending on operating conditions.
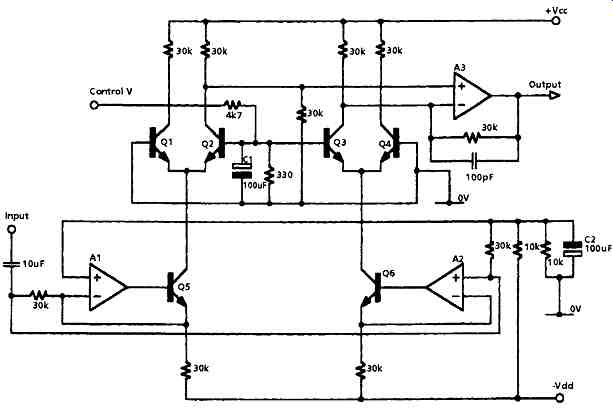
FIG. 19 Voltage controlled gain stage, due to PMI
If a step-type gain adjustment is acceptable, a simple option is shown in
the layout of FIG. 20, in which the output from a point on a resistive divider
chain is selected by one or other of a series of analogue switches. Since the
sequential operation of these switches can be done by the use of a voltage
controlled dot/bar driver IC, such as the LM3914, there would be little difficulty
or expense in assembling the hardware for this type of relatively low distortion
voltage operated volume control.
Digital Inputs
It has become customary for the manufacturers of compact disc players to provide
a direct digital output from the disc replay, usually as the RF signal derived
from the CD replay chain, shown, for reference, in FIG. 21. This RF output
will usually be taken at a point following the 14-8 decoder, but before the
Cross-Interleave Reed- Solomon code (CIRC) error correction stage shown in
the schematic replay layout of FIG. 21. This allows the manufacturer of specialist
digital to analogue signal-processing hardware to offer higher quality D/A
conversion systems, more jitter-free clock regeneration circuitry (which could
be very important in high precision D/A decoding), more competent error correction
regimes and better anti-aliasing filtration than might be offered in a budget-priced
CD player. It is argued that these latter signal handling stages probably contribute
more to the overall tonal quality of the CD player than the mechanical platform
itself or its control hardware.
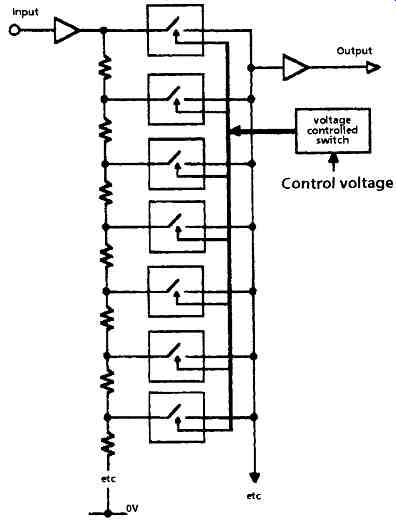
FIG. 20 Step-type gain control
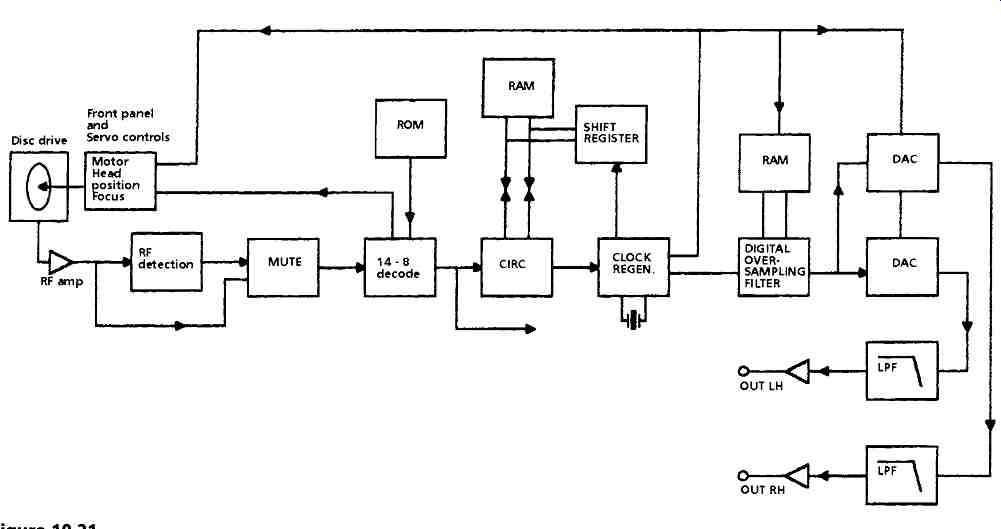
FIG. 21 CD replay schematic layout
In general, the RF output signal will be presented as a simple coaxial cable
output, but sometimes in top of the range units, this signal may also be presented
as an optical fiber output to avoid possible electrical interference or signal
degradation in the link between the CD player and the A/D decoder.
|